This article is published by Yue Yang in Science Magazine (Science 15 Jul 2016: Vol. 353, Issue 6296, pp. 300-305 DOI: 10.1126/science.aad4225). The authors share their findings about nucleosome remodeling and deacetylase complex (NuRD) and Chd4 in Neural Epigenetic Regulation through Chromatin Remodeling. Creative Biomart can provide some production for related research application.
[table caption=”Related Products” width=”800″ colwidth=”100|280|180″ colalign=”left|left|center|center|center”]
Cat. #,Product name,Source(Host),Species,Conjugate
CHD4-2793H,Recombinant Human CHD4 protein GST-tagged,Wheat Germ,Human,GST
CHD4-2434H,Recombinant Human CHD4 protein His-tagged,Insect cells,Human,His
CHD4-3379M,Recombinant Mouse CHD4 Protein,Mammalian Cells,Mouse,His
CHD4-27065TH,Recombinant Human CHD4,Wheat germ,Human,/
[/table]
Neuronal activity influences transcription in neurons, and hence regulates neural circuits. Activity-dependent genes are often rapidly transcribed and then rapidly inactivated. However, attention has focused on the induction of transcription rather than the biological roles and mechanisms of inactivation of activity-dependent transcription.
Epigenetic regulators, including adenosine 5′-triphosphate (ATP)-dependent chromatin-remodeling enzymes, are ideally suited to orchestrate the effects of neuronal activity on transcription globally. The ATP-dependent nucleosome remodeling and deacetylase (NuRD) complex triggers alterations of histone modifications, resulting in promoter or enhancer decommissioning and prolonged silencing of transcription.
To probe the role of the NuRD complex in dynamic regulation of transcription in the brain, we characterized the genome-wide occupancy of the core NuRD ATPase-encoding subunit, Chd4, in the mouse cerebellum. A substantial number of regions (9842) occupied by Chd4 in the cerebellum overlapped with transcription start sites (TSSs). Nearly all Chd4-bound TSSs (96%) harbored the histone modification H3K4 (histone H3 lysine 4) trimethylation (H3K4me3), which marks active and poised promoters, but not H3K27 trimethylation (H3K27me3), which marks inactive promoters (Fig. 1A). Chd4 binding at H3K4me3-enriched TSSs was diminished in the cerebellum in mice in which Chd4 was conditionally deleted in granule neurons (Fig. 1, A and B). Chd4 binding at H3K4me3-enriched TSSs in the cerebellum correlated tightly with acetylation of H3K9 and H3K14 (H3K9/14ac), which marks actively transcribed loci, and with gene expression (Fig. 1C). Thus, Chd4 occupies the promoters of most actively transcribed genes in the mouse brain.
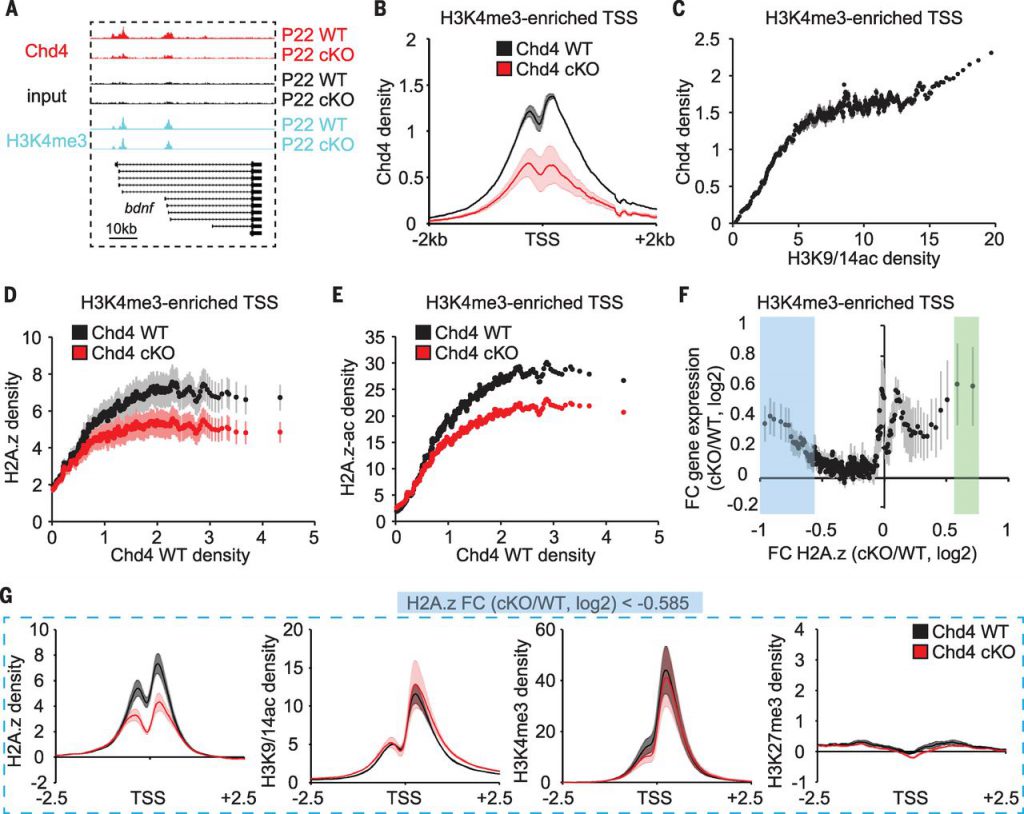
The NuRD complex triggers the sustained repression of only a small set of <200 genes in the cerebellum through diminution of H3K9/14ac and H3K4me3 at these promoters. We, therefore, reasoned that the NuRD complex might operate through another epigenetic mechanism to regulate the much larger set of Chd4-bound active genes. Exchange of the histone variant H2A.z is associated with regulation of transcription. H2A.z was enriched at 97% of Chd4-bound promoters in the mouse cerebellum. Chd4 knockout decreased H2A.z and acetylated H2A.z enrichment at promoters with high Chd4 occupancy (Fig. 1, D and E), but not at most enhancers in the cerebellum.
We next intersected RNA sequencing (RNA-Seq) and H2A.z chromatin immunoprecipitation (ChIP)–Seq analyses in the mouse cerebellum of conditional Chd4 knockout and control littermate mice. Although a small group of 121 up-regulated genes in conditional Chd4 knockout mice harbored increased H2A.z enrichment at their promoters, a much larger group of 1233 up-regulated genes displayed reduced H2A.z enrichment at TSSs (Fig. 1F). Gene ontology analyses revealed that genes with reduced H2A.z enrichment encoded proteins that function in intracellular signaling cascades, cell cycle control, and phosphorylation. Notably, there was little or no change in H3K9/14ac, H3K4me3, and H3K27me3 or in the density of histone H3 at the promoters of these genes upon Chd4 knockout (Fig. 1G). Together, these data indicate that the NuRD complex triggers the deposition of H2A.z at the promoters of a large group of actively transcribed signaling genes and inactivates their expression in the brain in vivo.
The identification of an epigenetic link from the NuRD complex to H2A.z at the promoters of signaling genes led us to investigate whether the NuRD complex regulates transcription dynamically in response to neuronal activity. Expression of the activity-dependent genes c-fos, nr4a1, dusp1, and nfil3 was increased in granule neurons of the rodent cerebellum upon membrane depolarization and rapidly inactivated 1 hour after cessation of membrane depolarization (Fig. 2A). Depletion of Chd4 or Mbd3, another subunit of the NuRD complex, impaired inactivation, but not reactivation, of activity genes in neurons after membrane depolarization (Fig. 2A). Thus, the NuRD complex appears to be required for inactivation of activity-dependent genes in neurons.
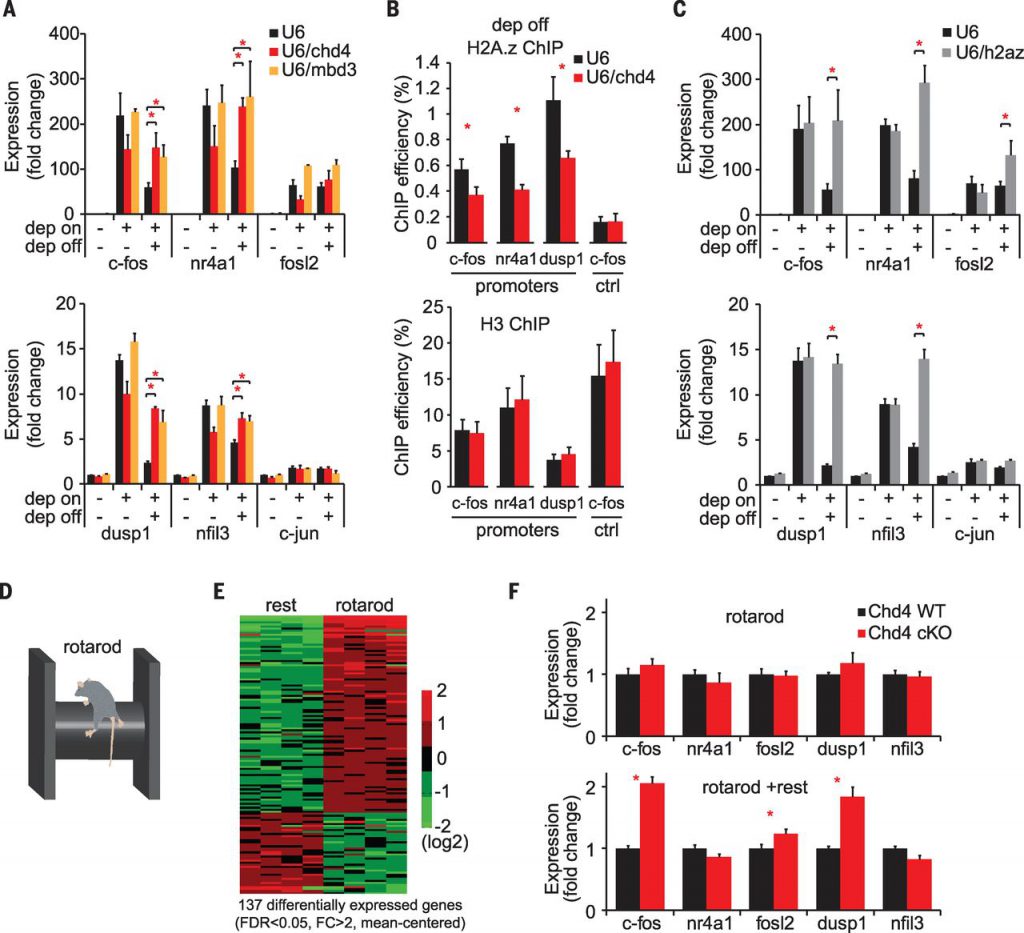
In chromatin immunoprecipitation followed by quantitative polymerase chain reaction (ChIP-qPCR) analyses, H2A.z increased at the promoters of the c-fos, nr4a1, and dusp1 genes in granule neurons during the inactivation phase after membrane depolarization. Depletion of Chd4 reduced H2A.z enrichment, but not histone H3, at the promoters of the c-fos, nr4a1, and dusp1 genes in neurons during the inactivation phase (Fig. 2B) but not the activation phase. Thus, the NuRD complex appears to specifically stimulate the loading of H2A.z at the promoters of activity-dependent genes during the inactivation phase of transcription.
Depletion of H2A.z by RNA interference (RNAi) in neurons increased expression of the c-fos, nr4a1, dusp1, and nfil3 genes during the inactivation phase of activity-dependent transcription, with little or no effect during activation or reactivation (Fig. 2C). Thus, the NuRD complex and H2A.z are required for inactivation of activity-dependent gene expression in neurons.
We next used a rotarod procedure to induce neuronal activity in the mouse cerebellum (Fig. 2D). RNA-Seq analyses from the cerebellum of mice running on a rotarod for 1 hour compared to mice housed in a cage revealed increased transcription of activity genes (Fig. 2E), and these genes were inactivated within 1 hour after rotarod activity stopped. Chd4 knockout increased the expression of c-fos, fosl2, and dusp1 in the cerebellum after cessation of rotarod activity but not during the activation phase of rotarod-induced transcription (Fig. 2F). Thus, the NuRD complex affects specifically the inactivation of activity genes in the brain.
We next tested whether NuRD-dependent inactivation of activity genes might regulate granule neuron connectivity in the cerebellum. We determined the stage of granule neuron maturation in vivo during which the NuRD complex regulates activity-dependent transcription. We used in vivo electroporation and translating ribosomal affinity purification to characterize gene expression in developmentally synchronized granule neurons in vivo (Sync-TRAP) (Fig. 3A). Sync-TRAP followed by qPCR or by RNA-Seq analyses of the cerebellum in mice 6 days after electroporation revealed that expression of granule neuron–specific genes was enriched, and led to the identification of chromatin regulators and ubiquitin ligases enriched in developing granule neurons (Fig. 3B). Sync-TRAP-Seq analyses in Chd4loxP/loxP mice electroporated with the recombinase Cre revealed that 86% of significantly differentially expressed genes were up-regulated upon conditional Chd4 knockout (Fig. 3C). Sync-TRAP-Seq and Sync-TRAP-qPCR analyses revealed that transcription of the activity-dependent npas4, nfil3, c-fos, and nr4a1 genes, but not of granule neuron–specific genes, was increased in granule neurons depleted of Chd4 in vivo (Fig. 3, D and E). Immunohistochemical analyses confirmed that c-Fos protein was up-regulated in Chd4-depleted granule neurons in vivo. Sync-TRAP-qPCR analyses also revealed that depletion of H2A.z increased c-fos gene expression in granule neurons in vivo. The NuRD complex and H2A.z thus appear to trigger the inactivation of activity-dependent genes in synchronously developing granule neurons in the mouse cerebellum.
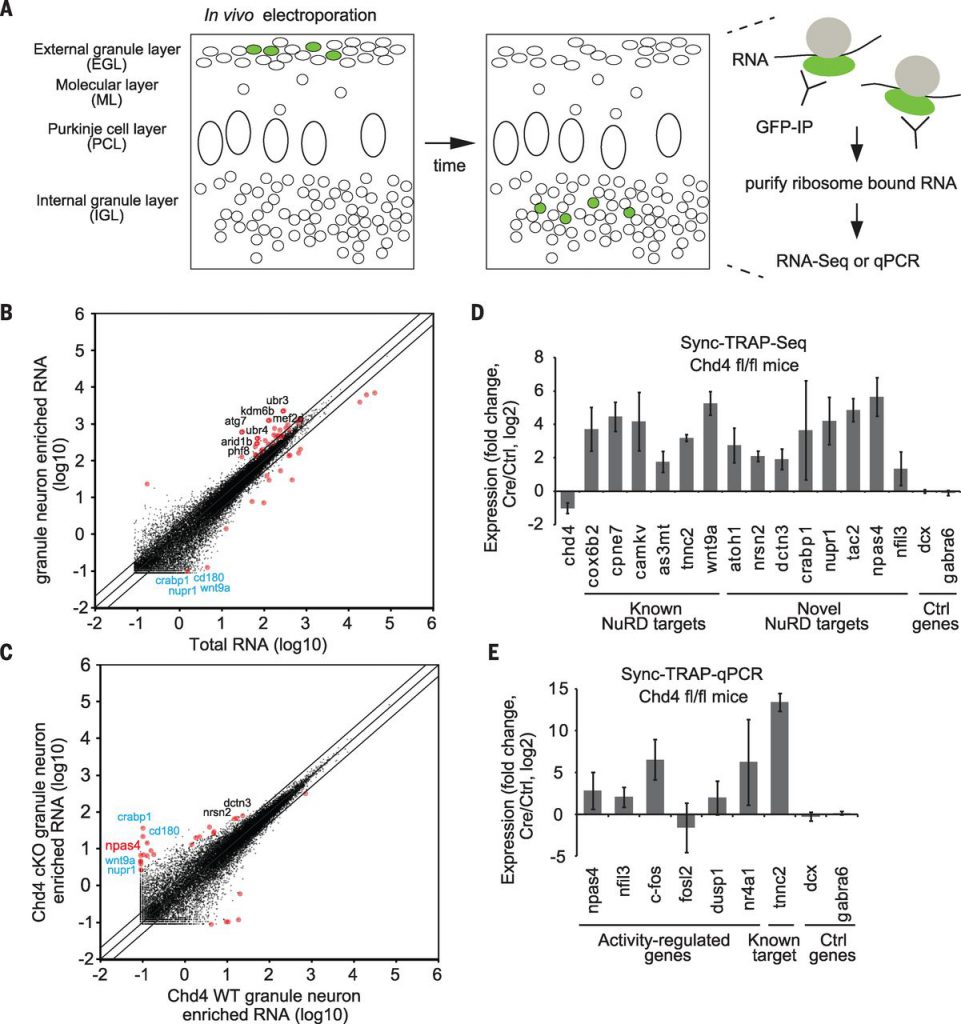
Because the NuRD/H2A.z epigenetic link regulates activity-dependent transcription in a temporal window of dendrite morphogenesis in the cerebellum, we asked whether NuRD-dependent inactivation of genes might influence dendrite patterning and connectivity. Granule neurons labeled by in vivo electroporation undergo distinct stages of dendrite morphogenesis in a synchronized manner in vivo (Fig. 4A). Depletion of Chd4 increased the total length of granule neuron dendrites and the number of primary dendrites during the period of pruning, with little or no effect on the development of granule neuron dendrites during earlier stages (Fig. 4B). Expression of NuRD-regulated activity genes also impaired dendrite pruning in vivo (Fig. 4, C and D). In contrast, upon expression of NuRD-repressed target genes not known to be regulated by activity or other cerebellum-enriched transcriptional regulators, only one increased dendrite length but not dendrite number (Fig. 4, C and D). These results indicate that NuRD-dependent inactivation of activity genes may drive granule neuron dendrite pruning in the cerebellum.
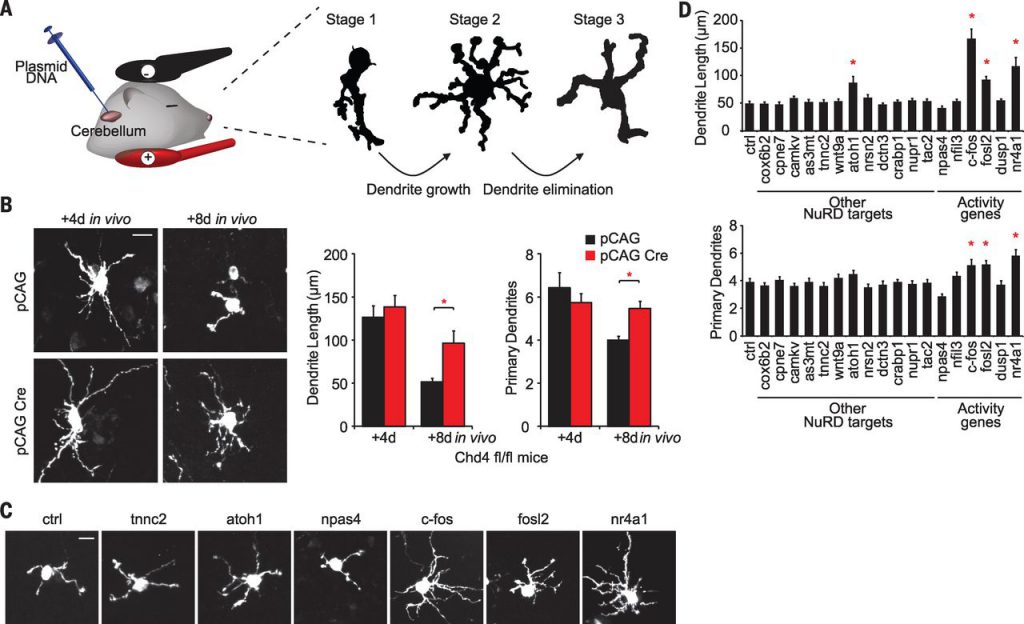
We next characterized the consequences of NuRD actions on responses of granule neurons in behaving mice. Mature granule neurons receive on average four mossy fiber inputs, which is optimal for sparse, lossless encoding of sensorimotor information. We electroporated mouse pups with a plasmid encoding the calcium indicator GCaMP6s together with an mCherry expression plasmid, and subjected mice to a motorized treadmill task (Fig. 5A). After habituation, two-photon imaging of lobule VI in the mouse cerebellum revealed that a set of GCaMP6s-labeled granule neurons was active during locomotion (Fig. 5, B and C). Conditional Chd4 knockout triggered a robust increase in the fraction of high-fidelity treadmill-responsive granule neurons and concomitantly reduced the fraction of unresponsive granule neurons (Fig. 5, C and D). Thus, inhibition of the NuRD complex leads to hyperresponsivity of granule neurons to sensorimotor stimuli.
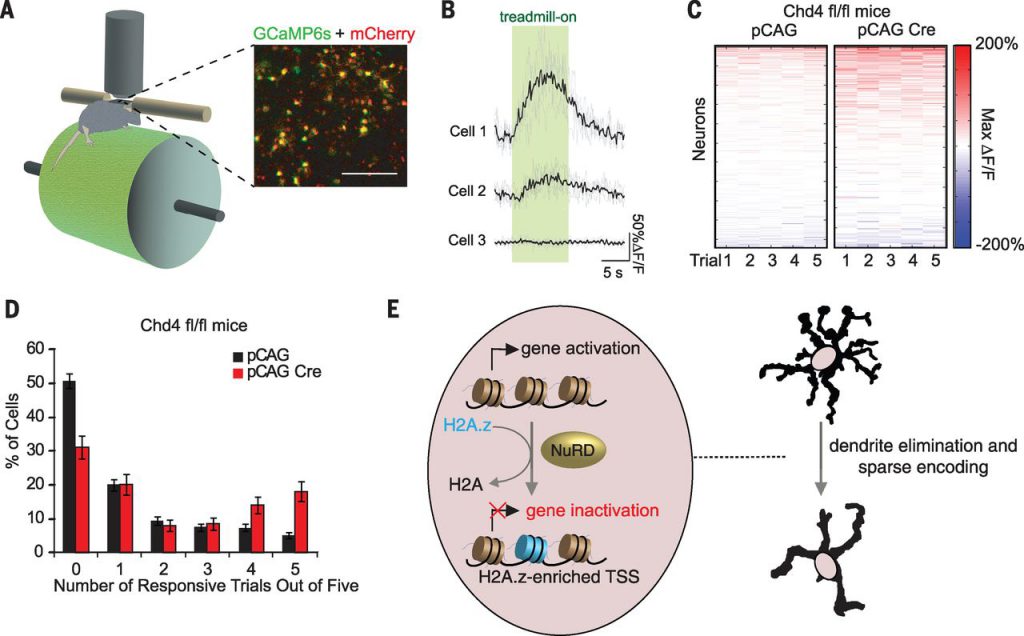
In behavior analyses, depletion of Chd4 in granule neurons impaired procedural learning, including in the accelerating rotarod and delay eyeblink conditioning assays, but had little or no effect on motor coordination as assessed in the DigiGait and open field assays.
Our study defines chromatin-remodeling events that inactivate activity-dependent transcription and control dendrite architecture and sensorimotor encoding in the brain (see model in Fig. 5E). Our findings suggest that inactivation of activity genes is essential for the maturation of granule neuron dendrite arbors and in the control of neural circuit activity in response to sensorimotor signals. We have uncovered the NuRD complex and H2A.z as epigenetic regulators that mediate the inactivation of activity genes in the brain. Thus, epigenetic mechanisms may have an active role in the inactivation of gene expression in the brain following neuronal activity.
For all details, please visit http://science.sciencemag.org/content/353/6296/300.