Here we share an article which summary a DNA edit method in living cells (Science 29 Jul 2016: Vol. 353, Issue 6298, pp. 444-445 DOI: 10.1126/science.aah4438). Creative Biomart provides kinds of molecular tools for the synthetic biology research applications using DNA.
Synthetic biology uses DNA programs to add new functions into living cells. By expressing transcription factors, microbes such as Escherichia coli can be made to perform complex computational logic and pass “memories” of selected events between generations. But DNA is more than just the source code for protein expression programs; DNA can be used as the storage medium for information. In synthetic biology, the use of DNA for in vivo information storage was first realized in 2009 with a synthetic genetic program that enabled E. coli to count events by using a recombinase to rearrange DNA in response to an input. With multiple recombinases, this technology could be used to store 1.375 bytes of information in a living E. coli. As reported by Shipman et al. on page 463 of this issue, and by Roquet et al. , in vivo encoding of information into DNA is pushed even further, using either genome editing to store dozens of bytes of data, or employing multiple recombinases to realize “state machines” inside living cells.
The clustered regularly interspaced short palindromic repeats (CRISPR)–Cas system is a prokaryotic adaptive immune system, in which CRISPR regions within the genome capture foreign DNA sequences during infections. These are later used by Cas9 to recognize and digest foreign DNA bearing the same sequence. The DNA sequences incorporated by CRISPR, called protospacers, form a memory of all previous infections encountered by the cell.
To create living cells engineered to record information into their DNA, Shipman et al. exploited the natural recording capacity of CRISPR-Cas using the native E. coli system to integrate multiple, user-defined protospacer sequences into CRISPR arrays. Using electroporation, bacteria take up oligonucleotides 35 base pairs (bp) in length and integrate this DNA as protospacers. Because the temporal order of protospacer acquisition events translates to a physical order in the CRISPR array, the record of what information (DNA sequences) was given to the cells at what time can be retrieved by sequencing the full CRISPR locus (see the figure). Shipman et al. integrated 3 sets of 5 oligonucleotide protospacers (15 elements) in this way.
To expand the data storage capacity of their system, Shipman et al. generated modified versions of Cas1 and Cas2 capable of integrating oligonucleotides in either forward or reverse orientations. Theoretically, this recording device can store between 20 and 100 bytes of DNA information in the genome of living E. coli. However, as CRISPR arrays are naturally capable of harboring hundreds of protospacers, it is likely that the storage capacity per cell could easily be increased.
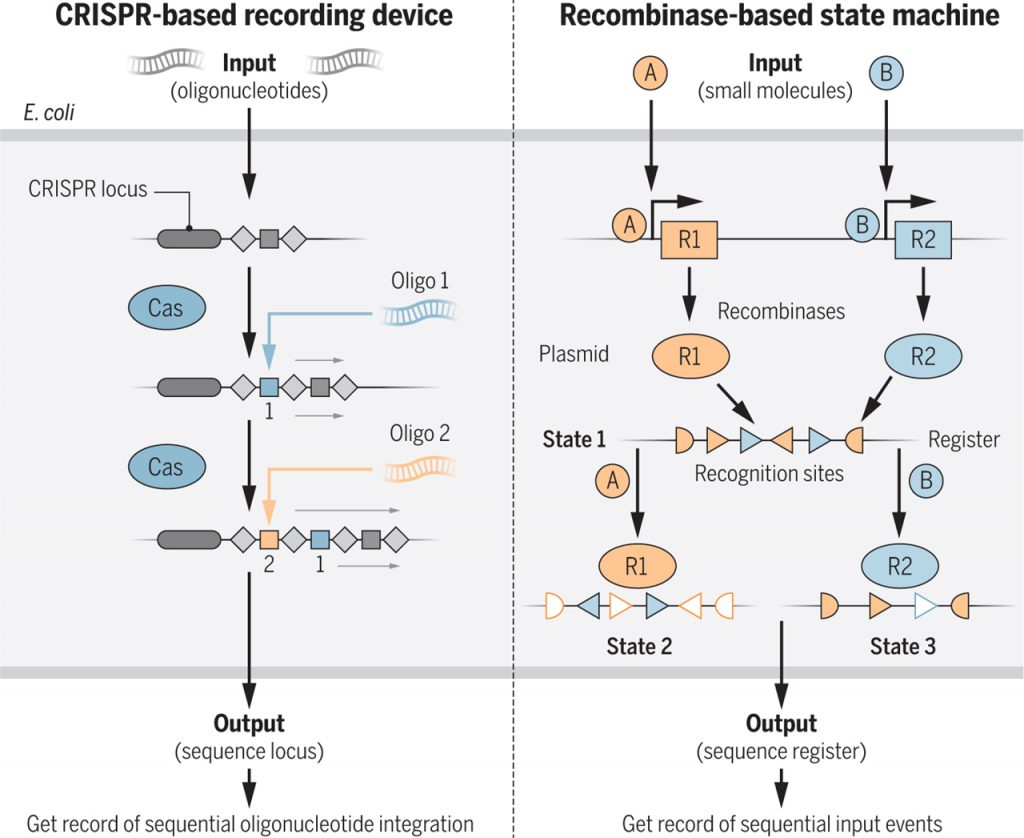
Although the method of rearranging DNA with recombinases is unable to store similar amounts of information in vivo, it can be much more readily exploited by having it interact with native and synthetic gene expression programs. Roquet et al. used recombinase-based memory to build a classic motif from computation—the state machine. State machines perform order-dependent input processing, so that at any point the system can be interrogated and the identity and order of inputs deduced. In response to external inputs, multiple recombinases recorded memory into a DNA “register,” a synthetic sequence hosted on a plasmid consisting of an array of nested recognition sites for the different recombinases. Depending on the inputs and thus on the order of activation of the different recombinases, the register is rearranged into distinct orientations (see the figure). With just three inputs controlling three recombinases, it is possible to build a 16-state machine in E. coli. Theoretically, with seven inputs and seven orthogonal recombinases, it would be possible to encode 13,700 distinct states.
To reveal the order and type of inputs received by any cell or population of cells, the DNA registers can simply be sequenced from the bacteria, or can also be quickly determined by quantitative polymerase chain reaction. Alternatively, the register sequences can be designed to contain genetic parts such as promoters and transcription factors. When these functional registers rearrange into certain states, they can trigger gene expression changes that lead to different E. coli phenotypes. Roquet et al. created a database of thousands of regulatory programs in which the cell’s behavior is tied to the state of the register. Using these biological outputs means that the state machine can lead the cells to take actions in response to the appropriate string of inputs.
Currently, the inputs used by both the CRISPR and recombinase systems are simple molecules. Roquet et al. used small molecules to activate recombinase expression, whereas Shipman et al. transformed synthetic oligonucleotides into cells. However, both studies suggest ways to feed in complex cellular phenotypes as inputs. By replacing recombinase regulation with alternative genetic control systems, Roquet et al. propose that state machines could monitor environmental signals or gene expression. Similarly, Shipman et al. suggest using their device to capture gene expression information by converting native messenger RNA transcripts into protospacers through the action of reverse transcriptase. As both Shipman et al. and Roquet et al. suggest, these strategies could be used to generate intrinsic devices within cells that autonomously record the timing of complex and inaccessible processes, such as gene dysregulation in cancer or developmental gene regulatory cascades. As McKenna et al. describe on page 462 of this issue, an autonomous method that continually mutates a genomic locus using CRISPR-Cas has provided valuable information for whole-organism genetic lineage tracing.
The methods used by Shipman et al. and by Roquet et al. represent new benchmarks in both in vivo information recording and biological computation, and show that DNA can be predictably edited over generations of living cells. Linking these DNA memories with the inherent power of cells to sense and act on their environments will no doubt lead to many exciting advances for synthetic biology.
You can reach this article by visit http://science.sciencemag.org./content/353/6298/444.full.
[table caption=”Related Products” width=”800″ colwidth=”120|180|180″ colalign=”left|left|center|center|center”]
Cat. #,Product name,Source(Host),Species,Conjugate
GTF2B-5018H,Recombinant Human General Transcription Factor II His-tagged,E. coli,Human,His
AES-28533TH,Recombinant Human AES His-tagged,E. coli,Human,His
ARFGAP2-30760TH,Recombinant Human ARFGAP2 His-tagged,E. coli,Human,His
ARID3A-28375TH,Recombinant Human ARID3A,Wheat germ,Human,/
[/table]