Cancer Immunology
Creative BioMart Cancer Immunology Product List
Immunology Background
Background
Cancer immunology is an intricate field that explores the complex interactions between cancer cells and the immune system with the goal of identifying biomarkers for cancer-immuno-diagnosis and developing novel cancer immunotherapeutic approaches.
Definition
Cancer immunology is the study of how the immune system interacts with cancer cells. The immune system, which consists of various cells, tissues, and organs, is primarily responsible for defending the body against pathogens and abnormal cells, including cancer cells. The goal of cancer immunology is to understand these interactions to develop novel therapeutic strategies that can enhance the immune system's ability to fight cancer.
Tumor Antigens
Tumor antigens are molecules expressed on the surface of tumor cells that can be recognized by the immune system, which may be released in the blood or remain on the cell surface. Antigens have been identified in most of the human cancers, including Burkitt lymphoma, neuroblastoma, melanoma, osteosarcoma, renal cell cancer, breast cancer, prostate cancer, lung cancer, and colon cancer. They are crucial for the immune system to distinguish between normal and malignant cells. Tumor antigens are typically categorized into two main types: tumor-specific antigens (TSAs) and tumor-associated antigens (TAAs).
Tumor-Specific Antigens (TSAs)
These antigens are unique to cancer cells and are not found on normal cells. They often result from mutations in the DNA of cancer cells that lead to the production of new proteins. Tumor-specific antigens can help the body mount an immune response against cancer cells. They may be used as possible targets for targeted therapy or immunotherapy to help stimulate the body's immune system to kill more cancer cells. Tumor-specific antigens can also be used in laboratory tests to help diagnose some types of cancer. Examples include mutated forms of the p53 protein and proteins produced by viral oncogenes in virus-associated cancers.
Tumor-Associated Antigens (TAAs)
Unlike TSAs, TAAs are present in both normal and cancer cells, but are overexpressed or abnormally expressed in cancer cells. In contrast to healthy cells, tumor-associated antigens are overexpressed in cancer cells and are often presented on the cell membrane via the major histocompatibility complex. Since these are normal, ubiquitous proteins, an immune response is often absent. However, if the antigen density on the cell membrane is high enough, the cancer cells can be recognized and destroyed by specific T cells. For example, tyrosinase is an enzyme expressed by normal melanocytes. It is highly overexpressed in malignant melanocytes. As a result, it is possible that patients with malignant melanoma may produce T cells that are specific for tyrosinase. In patients with breast cancer or pancreatic tumors, autologous lymphocytes were detected in the tumor tissue that reacted specifically against the mucin-1 overexpressed by these tumors.
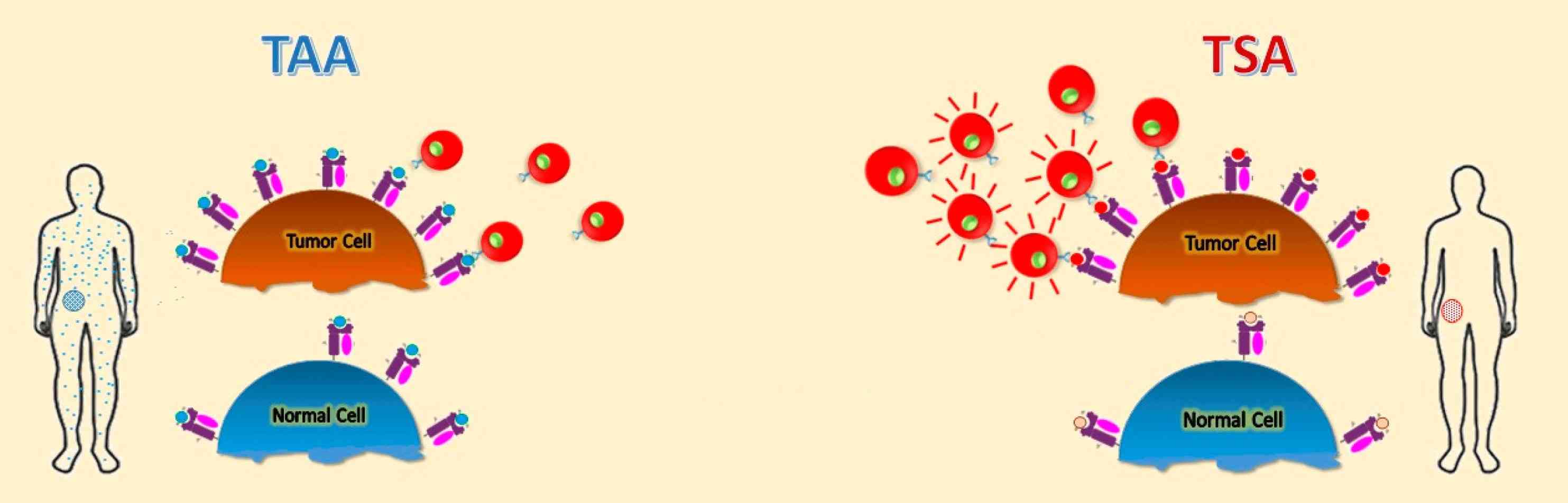
Recognition of these antigens by the immune system is critical for initiating an immune response against the tumor. However, cancer cells can employ various strategies to evade immune detection, such as downregulating antigen presentation or secreting immunosuppressive molecules.
Tumor Microenvironment
The tumor microenvironment is the dynamic and complex milieu surrounding tumor cells, including stromal cells, immune cells, blood vessels, extracellular matrix (ECM), and various signaling molecules. This environment is crucial for tumor initiation, progression, and response to therapy.
Stromal Cells
Stromal cells, including fibroblasts, endothelial cells, and pericytes, provide structural support and secrete factors that influence tumor growth. Cancer-associated fibroblasts (CAFs) are particularly important as they can remodel the ECM, promote angiogenesis, and secrete growth factors that support tumor cell survival and proliferation.
Immune Cells
The tumor microenvironment contains a variety of immune cells, including T cells, B cells, macrophages and dendritic cells. While some immune cells, such as cytotoxic T cells, can recognize and kill cancer cells, others, such as regulatory T cells (Tregs) and tumor-associated macrophages (TAMs), can suppress the immune response and promote tumor growth. The balance between these opposing forces is critical in determining the overall immune response to the tumor.
Extracellular Matrix (ECM)
The ECM is a network of proteins and glycoproteins that provides structural support to tissues. In the tumor microenvironment, the ECM is often remodeled to facilitate tumor invasion and metastasis. Components of the ECM can also influence cell signaling and modulate immune responses.
Signaling Molecules
Various cytokines, chemokines, and growth factors are present in the tumor microenvironment and play a key role in regulating cell behavior. These signaling molecules can promote inflammation, angiogenesis, and immune suppression, creating a favorable environment for tumor growth.
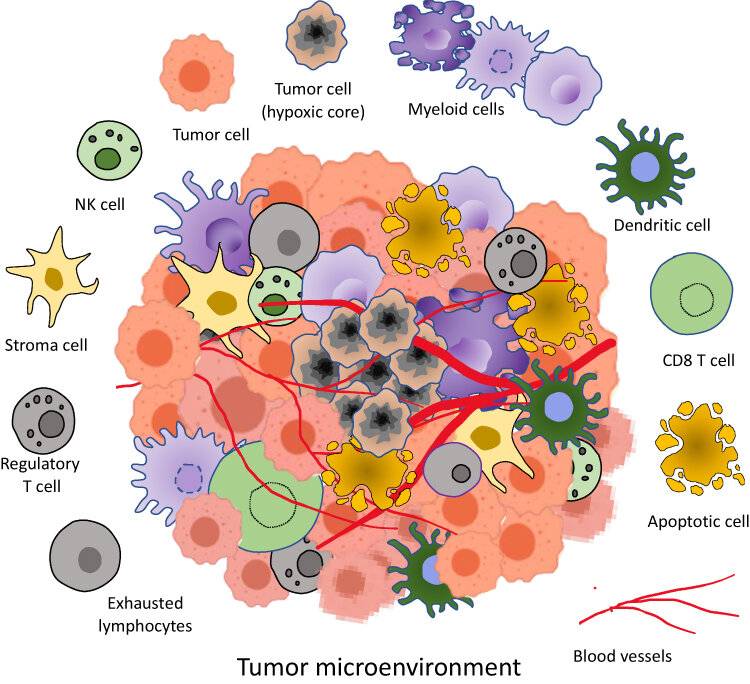
Immunotherapy
Immunotherapy is a groundbreaking approach to cancer treatment that harnesses the power of the immune system to fight cancer. Several types of immunotherapy have been developed, each with its own mechanisms and targets.
Checkpoint Inhibitors
Checkpoint inhibitors are drugs that block immune checkpoint proteins, which are molecules on immune cells that need to be activated (or inactivated) to start an immune response. Tumors can exploit these checkpoints to avoid immune attack. For example, the proteins PD-1 (on T cells) and PD-L1 (on tumor cells) interact to inhibit T cell function. Drugs like pembrolizumab (anti-PD-1) and atezolizumab (anti-PD-L1) block this interaction, allowing T cells to attack cancer cells.
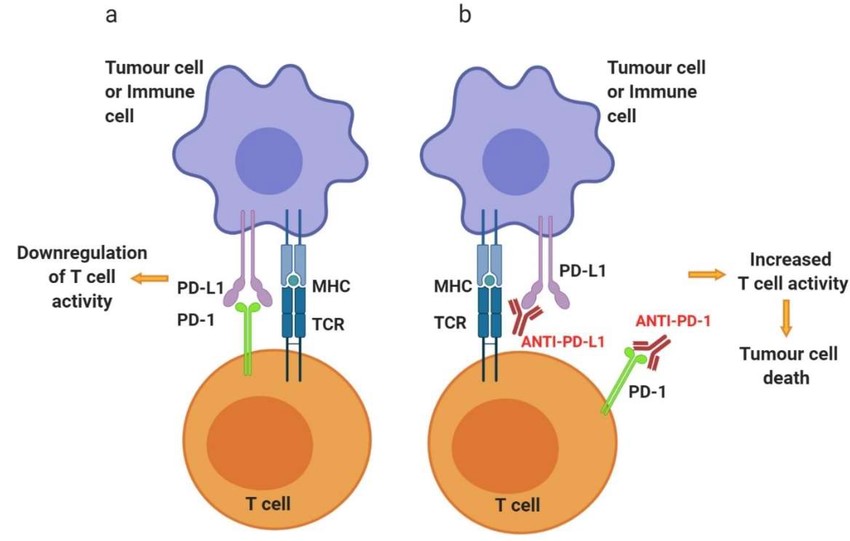
CAR-T Cell Therapy
Chimeric antigen receptor (CAR) T cell therapy, a patient's T cells are engineered to express CARs that recognize specific tumor antigens. These engineered T cells are then expanded in the laboratory and infused back into the patient. Targets of Car-T Cell Therapy include BCMA, CD19, HER2 etc. CAR T-cell therapy has shown remarkable success in treating certain types of blood cancers, such as acute lymphoblastic leukemia (ALL) and diffuse large B-cell lymphoma (DLBCL).
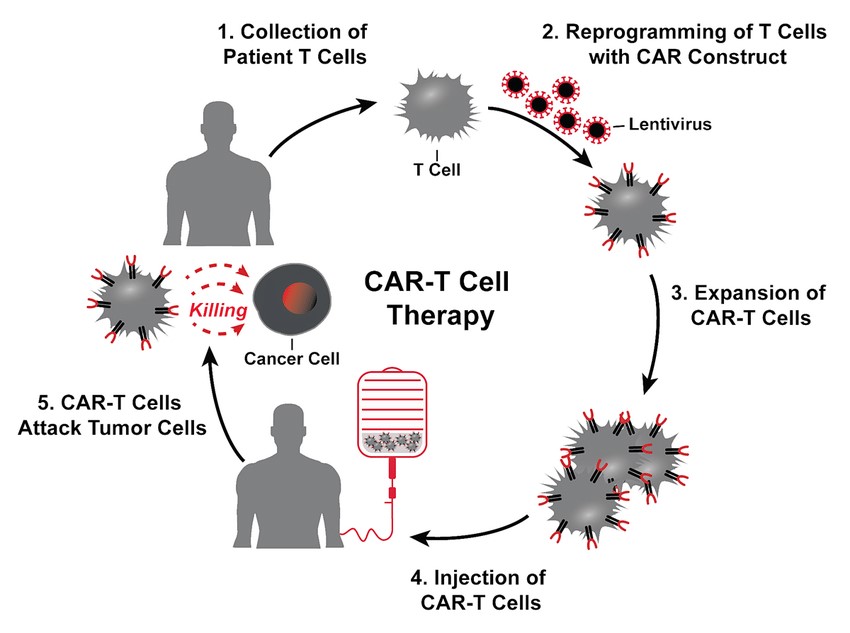
Cancer Vaccines
Cancer vaccines are designed to stimulate the immune system to recognize and attack cancer cells by introducing tumor antigens. Unlike traditional vaccines that prevent disease, cancer vaccines are typically therapeutic and used to treat existing cancers. In general, cancer vaccine platforms are classified into cell-based vaccines, peptide-based vaccines, viral-based vaccines and nucleic acid-based vaccines. An example is the HPV vaccine, which can prevent cervical and other cancers associated with human papillomavirus infection.
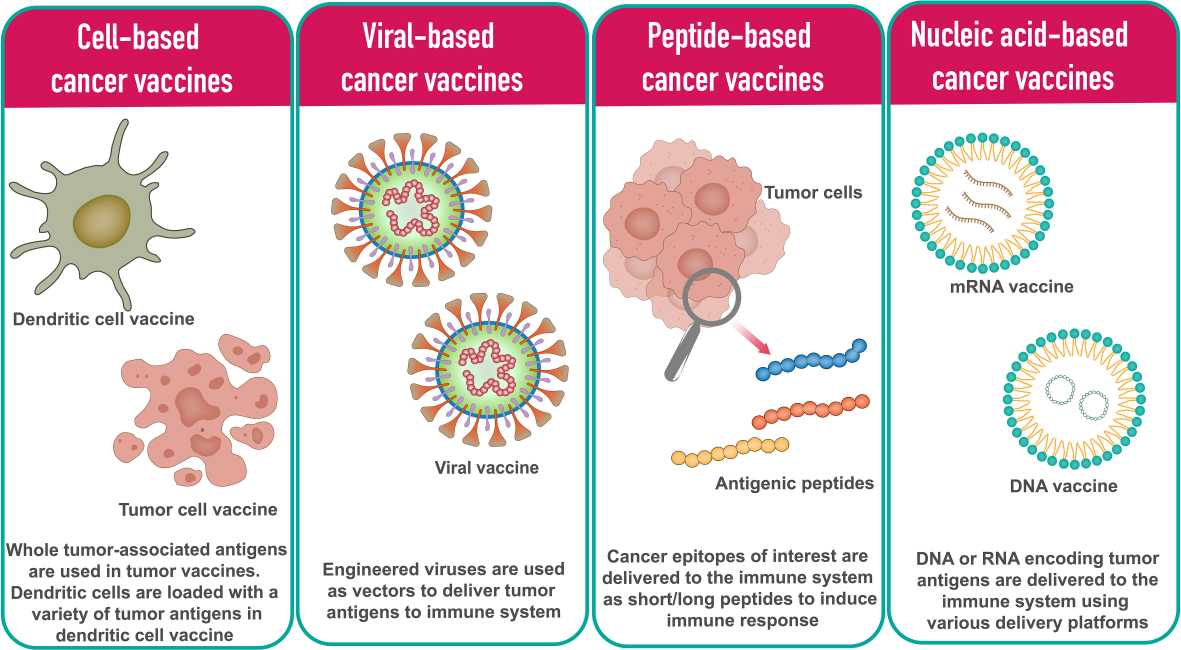
Adoptive Cell Transfer (ACT)
ACT involves collecting and using a patient's own immune cells to treat their cancer. This approach involves isolating tumor-infiltrating lymphocytes (TILs) from the patient's tumor, expanding them in the laboratory, and reinfusing them into the patient. This process enhances the body's ability to mount an immune response against the tumor.
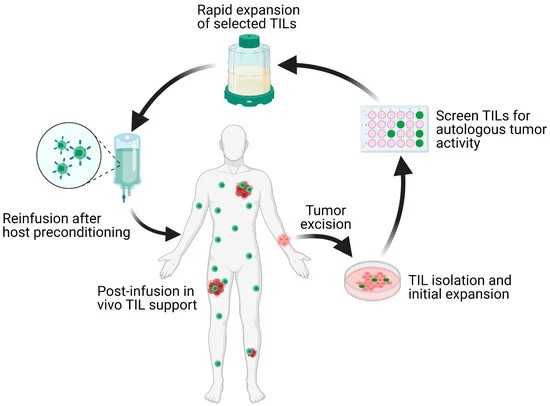
Case Study
Case 1: Ramos, C. A.; et al. Human papillomavirus type 16 E6/E7-specific cytotoxic T lymphocytes for adoptive immunotherapy of HPV-associated malignancies. J Immunother. 2013 Jan;36(1):66-76.
Vaccines are effective in preventing HPV-associated cancers. However, even though these tumors express viral antigens such as E6 and E7 proteins, they provide minimal benefit in treating existing cancers. This limited efficacy is likely due to negative signals in the tumor environment that prevent immune recognition and lead to T-cell inactivity in the body. In this study, the researchers hypothesized that by stimulating T cells outside the body, they could discover methods to reactivate and expand tumor-targeting T cells in HPV-positive cancer patients, which could then be used for adoptive immunotherapy.
E6/E7-specific T cells from 8/16 cervical and 33/52 oropharyngeal cancer patients were successfully reactivated and expanded (>1200-fold). Ramos and colleagues demonstrated that it is feasible to effectively generate T cell lines targeting HPV16 E6/E7 antigens from patients with HPV16-associated cancers.
To investigate the presence of HPV16 E6- and E7-specific (E6/7-specific) T cells in the peripheral blood of both healthy individuals and patients with HPV-associated cancers, the researchers generated a set of peptides (15-mers with 11 amino acid overlap) covering the unique 158 and 98 amino acid sequences of the E6 and E7 HPV16 proteins, respectively. The number of IFNγ-positive spot-forming cells (SFCs) was then measured as described in the Methods section. To determine whether pepmix-stimulated T cells could recognize endogenously processed viral antigens, they assessed their reactivity to autologous PHA blasts transduced with a retrovirus encoding either the E6 or E7 antigen. The results show that the cell lines producing IFNγ in an ELISpot assay with E6 or E7 pepmix showed the same reactivity to PHA blasts expressing E6 or E7, respectively (Fig. 7).
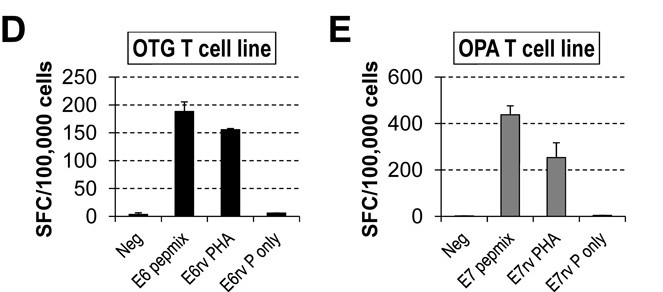
Case 2: Wang, B.; et al. Targeting of the non-mutated tumor antigen HER2/neu to mature dendritic cells induces an integrated immune response that protects against breast cancer in mice. Breast Cancer Res. 2012 Mar 7;14(2):R39.
In this study, the researchers evaluated the immunogenicity of a HER2 protein vaccine that directly targets DEC+ dendritic cells (DCs) in a murine breast cancer model. They genetically engineered the extracellular domain of HER2 into a DEC-specific monoclonal antibody (DEC-HER2). Mice of different genetic backgrounds were immunized with DEC-HER2 in combination with DC maturation stimuli (poly IC ± CD40 Ab). The results show that DEC-HER2 fusion mAb, but not Ctrl Ig-HER2, induces potent, broad and multifunctional CD4+ T cell immunity, CD8+ T cell responses and humoral immunity specific for HER2 antigen. Cross-reactivity with rat neu protein was also observed. In conclusion, immunization of mice with a HER2 protein vaccine targeting DEC+ DCs in vivo induced high levels of T and B cell immunity.
Specifically, C57BL/6 (H-2b) mice were immunized intraperitoneally with DEC-HER2 or Ctrl Ig-HER2. Poly IC and agonistic anti-CD40 mAb were used to mature DCs. In C57BL/6 mice, CD4+ T cells responded strongly to epitopes present in HER2 peptide pools 4 and 5 (Table S1 in Supplementary file 5 and Figure 3C of this article).
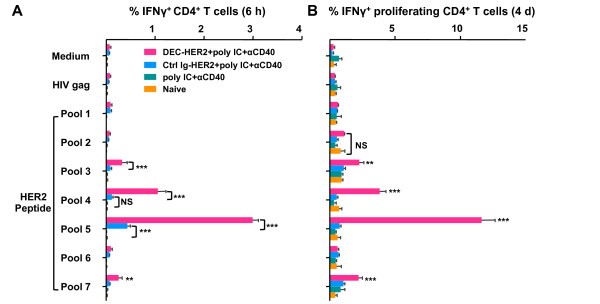
References
- Alard, Emilie, et al. "Advances in Anti-Cancer Immunotherapy: Car-T Cell, Checkpoint Inhibitors, Dendritic Cell Vaccines, and Oncolytic Viruses, and Emerging Cellular and Molecular Targets." Cancers, vol. 12, no. 7, July 2020, p. 1826. DOI.org (Crossref), https://doi.org/10.3390/cancers12071826.
- Allemailem, Khaled S., et al. "Innovative Strategies of Reprogramming Immune System Cells by Targeting CRISPR/Cas9-Based Genome-Editing Tools: A New Era of Cancer Management." International Journal of Nanomedicine, vol. 18, 2023, pp. 5531–59. PubMed, https://doi.org/10.2147/IJN.S424872.
- Buonaguro, Luigi, and Maria Tagliamonte. "Selecting Target Antigens for Cancer Vaccine Development." Vaccines, vol. 8, no. 4, Dec. 2020, p. 615. www.mdpi.com, https://doi.org/10.3390/vaccines8040615.
- Oliveira, André F., et al. "Review of PD-1/PD-L1 Inhibitors in Metastatic dMMR/MSI-H Colorectal Cancer." Frontiers in Oncology, vol. 9, May 2019, p. 396. DOI.org (Crossref), https://doi.org/10.3389/fonc.2019.00396.
- Picecchi, Dario, et al. "Towards Novel Reimbursement Models for Expensive Advanced Therapy Medicinal Products (ATMPs)." Swiss Medical Weekly, vol. 150, no. 5153, Dec. 2020, p. w20355. DOI.org (Crossref), https://doi.org/10.4414/smw.2020.20355.
- Qin, Shuyang S., et al. "Adoptive T Cell Therapy for Solid Tumors: Pathway to Personalized Standard of Care." Cells, vol. 10, no. 4, Apr. 2021, p. 808. www.mdpi.com, https://doi.org/10.3390/cells10040808.
- Ramos, Carlos A., et al. "Human Papillomavirus Type 16 E6/E7-Specific Cytotoxic T Lymphocytes for Adoptive Immunotherapy of HPV-Associated Malignancies." Journal of Immunotherapy, vol. 36, no. 1, Jan. 2013, pp. 66–76. DOI.org (Crossref), https://doi.org/10.1097/CJI.0b013e318279652e.
- Vishweshwaraiah, Yashavantha L., and Nikolay V. Dokholyan. "mRNA Vaccines for Cancer Immunotherapy." Frontiers in Immunology, vol. 13, Dec. 2022. Frontiers, https://doi.org/10.3389/fimmu.2022.1029069.
- Wang, Bei, et al. "Targeting of the Non-Mutated Tumor Antigen HER2/Neu to Mature Dendritic Cells Induces an Integrated Immune Response That Protects against Breast Cancer in Mice." Breast Cancer Research : BCR, vol. 14, no. 2, 2012, p. R39. PubMed Central, https://doi.org/10.1186/bcr3135.