What is FGF18 Protein
Having discovered in the mid-1990s, fibroblast growth factor 18 (FGF18) plays a crucial role in our understanding of the cellular and molecular biology. The FGF family, including the FGF18 protein, consists of 22 known members involved in a wide variety of physiological and pathological processes.
FGF18 is defined by its specific gene locus, positioned on the short (p) arm of chromosome 5 at position 22.1. It is encapsulated by a three-exon structure, and the encoded protein is a secreted product. FGF18 protein embodies a typical secondary structure composed of a mix of β-sheets and α-helices, signature to the FGF family.
Function of FGF18 Protein
FGF18 functions as a typical fibroblast growth factor, developing a crucial role in cell proliferation, differentiation, and migration by binding to the surface receptors of target cells. It holds a wide tissue distribution and is significantly involved in embryonic development, wound healing, and angiogenesis.
In terms of structure-function relationships, the FGF18 protein binds to FGF receptors (FGFRs). Specifically, it has high affinity towards FGFR3 and FGFR4, leading to the activation of various downstream signaling pathways such as the Ras/MAPK pathway, PI3K/Akt pathway, and PLCγ pathway.
FGF18 Protein Related Signal Pathway
The signaling pathway of FGF18 includes the interaction with an array of cellular components. It primarily involves binding to FGF receptors (FGFRs) on the cell surface, initiating a cascade of intracellular events. As mentioned earlier, FGF18 primarily conveys its bio-activities through the Ras/MAPK, PI3K/Akt, and PLCγ pathways, leading to controlled cell growth, survival, and differentiation.
This signaling is key in a wide array of cellular processes such as embryonic development, organogenesis, cell proliferation, angiogenesis, wound healing, and tissue repair. Additionally, FGF18 regulates chondrocyte proliferation and differentiation, contributing to long bone and joint development.
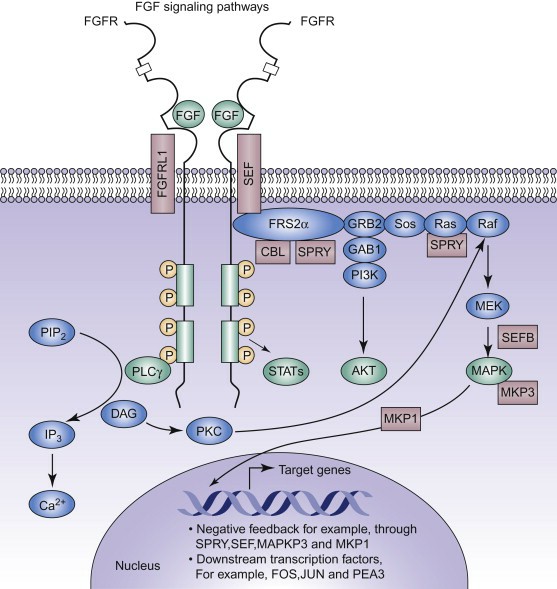
Fig1. FGF Signaling Pathway (José Marín-García, 2014)
FGF18 Protein Related Diseases
Despite FGF18's essential biological roles, it can also be linked to various disease occurrences. Overexpression of FGF18 has been connected to a variety of cancers, including lung, breast, pancreatic, and colon cancers. Here, it enhances cell proliferation and survival, contributing to tumor growth and invasion.
Pressing beyond the sphere of oncology, FGF18 plays a significant role in bone-related diseases. Its dysregulation can lead to disorders in bone and cartilage formation, such as achondroplasia and osteoarthritis, among others. FGF18's elevated expression has also been uncovered in osteosarcoma, the most common form of bone cancer.
FGF18 Protein's Applications in Biomedical
Echoing with its crucial roles in pathophysiology, FGF18 emerges as a promising therapeutic target for various diseases, primarily cancer and bone disorders. In cancer, the therapeutic potential is twofold: targeting FGF18 directly to inhibit its oncogenic function or exploiting it as a biomarker for diagnosis and prognosis assessment.
FGF18's indispensability in bone homeostasis points to its potential in treating bone-related diseases. Notably, therapeutic administration of FGF18 has shown promise in pre-clinical models of osteoarthritis by promoting cartilage repair.
Apart from direct targeting, the FGF18 protein manipulation might contribute to biomaterial and tissue engineering applications. Functionalization of artificial scaffolds with FGF18 might enhance their innative capabilities, promoting cell growth and tissue regeneration.
In conclusion, FGF18, with its association in diverse biological processes and medical conditions, establishes its significance in the biomedical field. Despite the challenges present, further research on this protein could potentially trickle down to significant clinical and therapeutic benefits.
Our Featured Products
Cat.No. | Product Name | Species | Source (Host) | Tag |
---|---|---|---|---|
FGF18-183H | Recombinant Human FGF18 protein, His/S-tagged | Human | E.coli | His/S |
FGF18-12867H | Recombinant Human FGF18, GST-tagged | Human | E.coli | GST |
FGF18-224H | Active Recombinant Human Fibroblast Growth Factor 18, HIgG1 Fc-tagged | Human | CHO | Fc |
FGF18-171H | Recombinant Human Fibroblast Growth Factor 18 | Human | E.coli | N/A |
FGF18-223H | Active Recombinant Human Fibroblast Growth Factor 18, MIgG2a Fc-tagged | Human | CHO | Fc |
GF18-4817HF | Recombinant Full Length Human FGF18 Protein, GST-tagged | Human | In Vitro Cell Free System | GST |
FGF18-031H | Active Recombinant Human FGF18 Protein | Human | E. coli | |
FGF18-2946H | Recombinant Human FGF18 Protein, His (Fc)-Avi-tagged | Human | HEK293 | His (Fc)-Avi |
Reference
- José Marín-García M.D., Molecular Determinants of Cardiac Development, in Post-Genomic Cardiology (Second Edition), 2014